Vitreoretinal adhesion is known to be an important factor in many ocular disorders as well as in traumatic eye injury. Detailed analyses of the structure of the vitreoretinal interface have been performed and it’s theorized that collagen fibers are the predominant source of adhesion. In some regions of the eye (vitreous base, posterior pole), the collagen fibers have been shown to traverse the vitreoretinal interface perpendicular to the surface of the retina. However, in other regions (equator), the collagen fibers run parallel to the surface and it’s thought that other collagen proteins lie between the vitreous cortex and retina acting as a type of ‘glue’ between the structures. This creates a regional variation in vitreoretinal adhesion. Furthermore, as an eye ages, the collagen breaks down. This would result in changes in vitreoretinal adhesion wiht age. To test this, we are currently quantifying collagen at the vitreoretinal interface and correlating to measurements of adhesion on a macroscopic and microscopic scale.
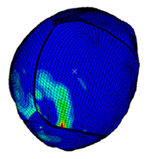
Traumatic brain injury (TBI) is the most common cause of death in childhood. The predominant etiologies of TBI in young children are motor vehicle accidents, firearm incidents, falls, and child abuse. Unfortunately, falls are the leading cause of non-inflicted head injury in infants less than a year old, and are also the most common history provided by caretakers suspected of child abuse. The objective of this research proposal is to provide clinicians with a biomechanics-based tool to aide in the diagnosis of inflicted or non-inflicted trauma with a history of a low height fall.
Using dynamic material property data of infant cranial bone and suture, experimental studies of rapid head rotation, instrumented anthropomorphic surrogate simulations and finite element modeling, we plan to recreate several real-world cases of children < 1 year old experiencing a low height fall. By correlating the mechanical stresses and strains from these recreations with the injuries we can identify the best biomechanical parameters for predicting skull fracture and intracranial hemorrhage, and determine stress and strain thresholds for injury.
Supported by the Department of Justice
The goal of this proposal is to reduce the risk of traumatic brain injury (TBI) through smart technology that collects sensory data to predict and characterize impacts in real-time, optimizes protective mechanisms based on impact characteristics (e.g., direction, velocity), and transmits final impact attributes to a database for further analysis and injury risk prediction. This technology will substantially improve TBI prevention and diagnosis in motor vehicle crashes, sports, and industrial accidents. The unique technology will leverage musculoskeletal and biomechanical computational models linking head linear and angular acceleration to brain deformation and injury. To accomplish this goal, fundamental research efforts include (1) real-time situational monitoring to predict when and how dangerous impacts are about to occur and (2) active prevention mechanisms in the form of anticipatory muscle activation and event-specific force damping bladders to reduce the risk of TBI due to impact. Initial evaluation of the technology will occur in a sports setting, but the integrated system will be widely applicable to multiple etiologies of TBI.Supported by the National Science Foundation
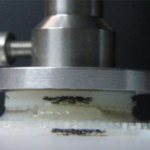
Supported by the Knights Templar Eye Foundation

Supported by Department of Defense Vision Research Program
We hypothesize that children can sustain head injury from cyclic loading without impact to the skull. No data exists to test this theory. To date, we have designed a well-defined model of head trauma due to a single, rapid head rotation, but the device does not allow for repetitive loading. We have now designed and built a mechanical system capable of producing 3Hz of back and forth head rotation at acceleration loads similar to those measured in surrogate simulations of shaking. With this device we propose to measure the effect of a single cyclic head rotation versus cyclic head rotations on tissue injury, identify the effect of cyclic head injury on behavior and cognition, use finite element analysis to identify tissue strain from cyclic loading, and determine injury tolerances due to cyclic head rotation in children.
Supported by the Primary Children’s Medical Center Foundation
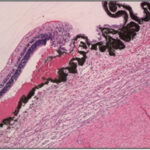